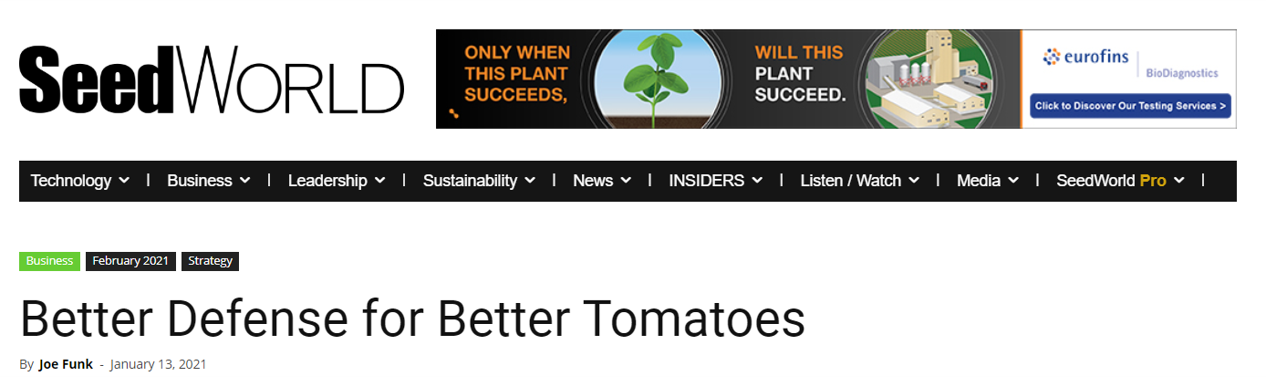
As Tomato Brown Rugose Fruit Virus infects tomato and pepper plants across the globe, seed companies are working on breeding a resistant tomato.
Thousands of years ago, when farmers in South America began domesticating tomato’s wild ancestors, Solanum pimpinellifolium, they were working with weedy-looking plants that produced small albeit intensely flavored fruit. Over time, through continued selection, tomatoes evolved into the larger, modern cultivated tomato. What modern tomatoes gained in size, they lost in disease resistance and ability to withstand abiotic stress. For more than 100 years, plant breeders have been on a continual trek to improve tomato yield and disease resistance.
Rich genetic and genomic resources were available to tomato breeders even before the inception of the tomato’s genomic sequencing projects. Large tomato germplasm collections have long been established in several locations. More than 75,000 tomato accessions are conserved in genebanks around the world. One of the largest is the USDA genebank at Geneva, NY.
Sequencing of the tomato genome was initiated in 2005 as a multinational effort between 14 countries and completed in 2012. These efforts have been greatly accelerated with the application of artificial intelligence, machine learning and advanced algorithms to analyze large quantities of genetic and phenotypic data.
Tomato Pan-Genome
Researchers from the Boyce Thompson Institute in 2020 created a high-quality reference genome for wild tomatoes to produce a more complete and useful tomato pan-genome. They discovered sections of the genome that underlie fruit flavor, size and ripening, stress tolerance and disease resistance. Thanks in part to cutting-edge sequencing technologies that can read very long pieces of DNA, the reference genome is more complete and accurate than the existing database.
Older sequencing technologies that read shorter pieces of DNA identifies mutations at the single-base level. However, they are not good at finding structural variants such as insertions, deletions, inversions or duplications of large chunks of DNA.
“Many known traits of the tomato are caused by structural variants,” says pan-genome project leader Zhangjun Fei. “Structural variants are understudied because they are more difficult to identify. That is why we focused on them.”
In 2019, an international group of researchers constructed a tomato pan-genome using genome sequences from 725 phylogenetically and geographically representative varieties. This database includes 4,873 genes not found in the original reference genome. Present/absent variation analyses revealed substantial gene loss and intense negative selection of genes and promoters during tomato domestication and improvement.
Fei’s group compared their wild variety genome to the genome of Heinz 1706, a cultivated variety included in the construction of the original tomato genome. The comparison found more than 92,000 structural variants. Many of these structural variants are of potential interest to plant breeders. The variants pertain to numerous disease-resistance genes as well as to genes involved in fruit size, ripening, hormonal regulation, metabolism, and the development of flowers, seeds and leaves.
Once a genomic region is found, molecular geneticists carefully examine the region for any variation that could contribute to that trait. Some of the apparent causes are single nucleotide polymorphisms (SNPs), but thus far, most have been caused by a structural variant. Knowing the structural variants in the genome facilitates the discovery of novel genes that control traits such as resistance or weight, shape and flavor.
“A breeder wants to know exactly where these variants occur in order take it one step further to figure out what the change is,” says University of Georgia plant breeder and horticulture researcher Esther van der Knaap. “The genome is linear in higher organisms. The genes are in a certain order in most tomato plants. In other tomatoes, there is a region of about 300KB that is inverted. We don’t know how that happened, but it is inverted. As tomato plants evolved, segments of DNA were deleted, duplicated or rearranged. These structural variations in genomes underpin the vast diversity among tomatoes.
“We cross tomato varieties all the time. One pairing might make bigger fruit while another might make more fruit, but there are hundreds of populations. We would need 10 times the greenhouse space to evaluate them all. We have to be smart about it and choose which crosses are going to be the most informative, which ones are going to give us new genes we don’t know about yet. With the knowledge we create, we can select traits at the genome level that we know, as breeders, will result in a variety with superior qualities.”
A new analysis of genetic variation among tomatoes by van der Knaap’s team uncovered 230,000 previously hidden large-scale differences in DNA between varieties. All this variation provides data that can be analyzed by a molecular geneticist to identify markers. It is useful for developing new varieties without large, time-consuming field trials. One of the most pressing challenges is to use this new-found genetic variation to develop varieties with genetic resistance to the Tomato Brown Rugose Fruit Virus (ToBRFV).
Tomato Brown Rugose Fruit Virus
An outbreak of a new disease infecting tomatoes occurred in 2014 in Southern Israel. It was identified as Tomato Brown Rugose Fruit Virus (ToBRFV) and has spread to most major tomato production regions. One of the most distinguishing and problematic characteristics of ToBRFV is its ability to overcome genetic resistance in tomatoes, including the well-known TM-22 gene. The disease can cause a 100% yield loss. In the absence of genetic resistance or other effective mitigation measures, the virus is of great concern to the global production regions. “Rugose” refers to the rough and wrinkled leaves present on infected plants.
Some private companies, including Bayer, BASF, Syngenta and Enza Zaden are working on breeding genetic resistance. During the last two months of 2020, these companies all announced making progress toward developing tomato varieties with a significant level of ToBRFV resistance.
Enza Zaden has defined two levels of protection: high resistance (HR) and intermediate resistance (IR). The company describes plant varieties that highly restrict the growth and development of ToBRFV under normal pathogen pressure as high HR. These plants may exhibit some symptoms or damage under heavy pathogen pressure. IR varieties will show less severe damage than susceptible plants when grown under similar environmental conditions.
Syngenta Vegetable Seeds announced it had launched its first commercial ToBRFV resistant variety to be available in early 2021. The new variety will be released in areas where growers face severe disease pressure from the virus and will support against crop losses.
“With fast development and use of molecular markers, Syngenta is able to rapidly make use of this resistance, deploying it in a large range of varieties; spreading the resistance in our germplasm,” says Pilar Checa, Global Breeding Lead for Tomato. He explains that before the utilization of molecular markers, it could take up to 10 years to develop a resistant variety.
Bayer announced the final large-scale pre-launch trials of Roma-type ToBRFV-resistant tomatoes. The trials, which begin in September in Mexico, will include two varieties of Roma-type tomato that claim IR to ToBRFV. The type of IR Bayer has seen in these varieties is commonly referred to as symptomless or asymptomatic carriers. Despite the presence of virus particles, these plants show little or no symptoms of the virus in the leaf and/or fruit if the crop becomes infected by the virus. Bayer anticipates its seed products will be available for commercial sale in Mexico in 2021, to be followed later by offerings in other important markets around the world.
NRGene and Philoseed, both located in Israel, are collaborating to use artificial intelligence and machine learning to identify and develop genetic resistance. NRGene’s advanced multi-purpose breeding platform is an artificial intelligence (AI) cloud-based solution for managing the full genomic diversity of species. It can analyze unlimited volumes of genomic data, enabling scientists and breeders to easily relate genomic sequences with beneficial traits, making a genomic selection and trait mapping much more productive. Data use is accelerated, making breeding both faster and more cost-effective.
Philoseed has the expertise and experience in combining its unique tomato genetic material to enable the identification of extremely strong resistance to the ToBRFV. Crossing this resistance source with its advanced commercial lines is expected to give Philoseed a strong presence in the markets in which it is active.
“Everybody wants a better tomato with more yield,” says Dr. Ehud Kopeliovitch, breeding manager at Philoseed and a global renowned tomato breeder. “Nutritional value and functional food values are the issues companies are requesting. Some want longer shelf life, improved flavor: they want to put everything together. In the last five years, the main concern among plant breeders has been resistance to the Tomato brown rugose fruit virus. Everybody is looking to solve that problem all at once with better genetics, not by breeding to make incremental gains. Genetic resistance is at the frontal focus of the industry.”
If someone were to ask Dr. Ben Gradus, NRGene Genomics solutions specialist at NRGene to describe what makes a good variety, he would tell you it is not his job to describe a good tomato. On the other side, when a plant breeder looks at a variety, they can see and evaluate phenotypic traits. They will tell whether the vine looks good, the tomato looks good and if the tomato has strong resistance to a new virus. Neither of them has the complete picture. It is when the plant breeder takes an ideal plant to the genomics solutions specialist is when the discovery begins to happen.
Gradus can use his tools to look into the genetics of a plant and get a picture of the genetic composition of an ideal variety. He has no need to check whether the breeder is wrong or right. Instead, they can now say, “Wow, we know the sequence of the genetics we want in our new plant.” Then, the next time the breeder shows a plant, they can analyze it and tell whether it is the one they want. They can tell if it is similar to what they want or how close it is to what we want. When we put those things together, we can get the benefits of the synergy between the two disciplines.
“We believe if we pooled the capacity of NRGene and Philoseed, we could get a very strong tool with the leverage to break the new ToBRFV virus which is probably the most concerning issue in the worldwide tomato industry today,” he says.
NRGene is using state-of-the-art genetic computational technology to introduce new genetic material into several elite varieties in far less time. The process uses a minimum number of generations to integrate a trait. Fewer plants are required to discover molecular markers for a given trait, often with 100 progenitors or less to develop molecular markers for disease resistance or any other trait. This reduces the amount of needed greenhouse space. Cloud computing has made it possible to manage and transfer the large amount of data needed to develop proprietary molecular markers.
In the past year, the diminishing need for field observation has eliminated many of the geographical barriers and restrictions imposed by COVID-19 lockdowns. While other companies were restricted and unable to make field observations, NRGene’s work continued and was largely unimpeded by lockdowns.
“We take the benefits of cloud computing, artificial intelligence and machine learning together with plant breeding to get results faster than we could do if we had to always rely on field observations. NRGene is now in a position to be looking at bringing projects first to market,” says Gradus.
This progress is all without the use of biotechnology methods such as transgenic modification (GMO) or CRISPR intervention. The progress involves accelerated classical breeding and is made more accurate by big data and molecular marker development.